Electrical Shock Care
Historically, the electrical injury was considered a form of thermal burn injury mediated by Joule Heating. However, over the past twenty years, medical research has revealed that this is just one component of a complex multi-system injuries. Electrical trauma produces a pattern of injury that depends on three main factors: the intensity and frequency of the current, the anatomical path that the current takes through the body, and the duration of current exposure.
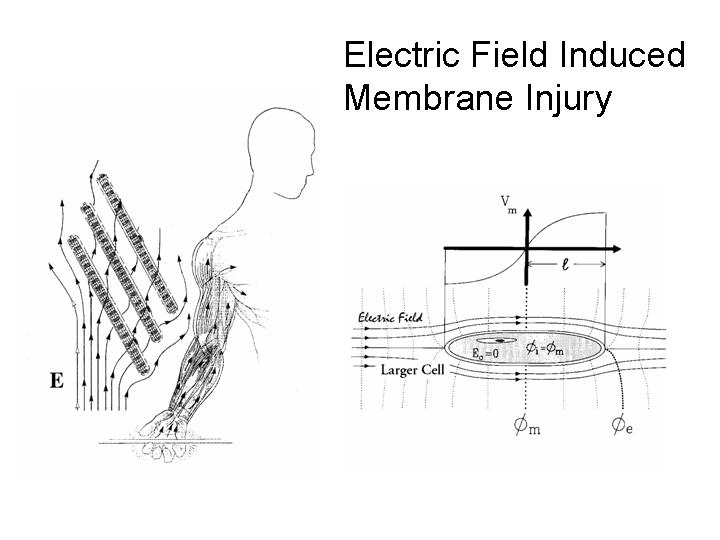
While much of the research attention is focused on complex, high-energy electrical shocks, the most common electrical shocks are caused by contact with low energy power sources such as household electrical power circuits. Aside from the small skin burn-puncture contact wounds, the harmful effects of low energy electrical shocks are linked to direct electrical effects on tissues, such as interference with normal electrophysiological signaling function of muscles and nerves and structural disruption of muscle and nerve cell membranes through a process called electroporation.
Electric shocks lead to molecular alterations (i.e electroporation and electro-conformational coupling) that occur on a submillisecond time scale. These effects alter the structure and compromise the functional integrity of the cell plasma membrane. Skeletal muscle and peripheral nerve cells are particularly vulnerable to direct electrical force effects because of their relatively large size compared to other tissue cells. The electric field required to electroporate skeletal muscle and peripheral nerve cells is typically 100-1,000 fold smaller than that required to electroporate other, much smaller, cell types. Engineering models of human high-voltage electrical shock suggest that the tissue electric field strength can be of sufficient magnitude in the extremities to electroporate skeletal muscle and peripheral nerve cell membranes during the shock. They can also cause electro-conformational denaturation of membrane proteins. When any form of trauma disrupts cell membranes, the cell's metabolic energy will be quickly exhausted, leading to a metabolic arrest and eventually to lost viability.
Prolonged contacts, on the scale of seconds, cause thermal damage to deep tissues that begin to dominate the injury accumulation processes. If the high-energy electrical source contact involves an arc-fault, thermo-acoustic blast forces can contribute to the injury. Lightning strike victims experience similar thermos-acoustic blast (i.e., "thunder") forces that contribute to the injury process.
UNDERSTANDING ELECTRICAL INJURY
Electrical injury can result from direct contact with any source of electricity. While some electrical shocks do not result in significant external burns, they can still result in serious internal damage, producing a complex pattern of injury and clinical manifestations. An electrical injury's exact pathophysiology is very difficult to predict due to the large number of variables that cannot be measured or controlled when an electrical current passes through tissue.
Accidental contact with exposed parts of electrical appliances or wiring, flashing of electric arcs from high-voltage power lines, lightning, machinery, or occupational-related exposures, or poking metal objects into an electrical outlet are a possible cause of electrical injury. High-voltage electrical trauma mainly observed in electrical workers produces some of the most devastating of physical injuries. Repeated removal of the damaged tissue and extensive rehabilitation are common, while limb amputation rates for victims who experience direct electrical contact can be as high as 75%. In general, most victims who survive high-voltage electrical shock are left permanently disabled.
Away from the workplace, most electrical injuries are due to either indoor household low-voltage (less than 1000 V) electrical contact or outdoor lightning strikes.
Domestic household 60-Hz electrical shocks are common and usually result in minor peripheral neurological symptoms or occasionally skin surface burns. However, more complex injuries may result depending on the current path, particularly following biting or chewing on household appliance cord outlets or disclosures in small children. Compared to a high-voltage shock that usually is mediated by an arc, low-voltage shocks are more likely to produce a prolonged, "no-let-go" contact with the power source. An involuntary, current-induced muscle spasm causes this "no-let-go" phenomenon.
Even without visible burns, survivors of electric shock may face long-term discomfort and muscular pain, headache, fatigue, problems with peripheral nerve sensation and conduction, inadequate coordination and balance, and other additional symptoms. Electrical injury often leads to neurocognitive function problems, affecting the speed of mental processing, attention, concentration, and memory. The high frequency of psychological problems is very well established and may be multifactorial in etiology. As with any traumatic and life-threatening experience, an electrical injury may result in post-traumatic psychiatric disorders, which can have an impact that is equally life-changing to that caused by a major physical deformity. Experienced consultants and a supportive environment are required to facilitate rehabilitation, return to gainful employment, and normal family and social functioning.
CELLULAR BIOPHYSICS
THEORETICAL AND EXPERIMENTAL ASPECTS OF THE EFFECTS OF ELECTRICAL FIELDS ON MEMBRANE PROCESSES
Our group's focus is on understanding how time-dependent electric fields (e.g., oscillating membrane potentials observed in many systems) influence membrane proteins' behavior. In particular, we use the theory developed to probe the kinetics and mechanisms by which biological signaling and energy transduction are accomplished. At present, much of our work focuses on fundamental aspects of the statistical mechanics of diffusive and reactive processes in the presence of fluctuating fields and potentials. There is no solid basis for this topic in the physical and mathematical literature, but this is an area that will be extremely important for understanding how membrane proteins accomplish their energy—and signal-transducing roles.
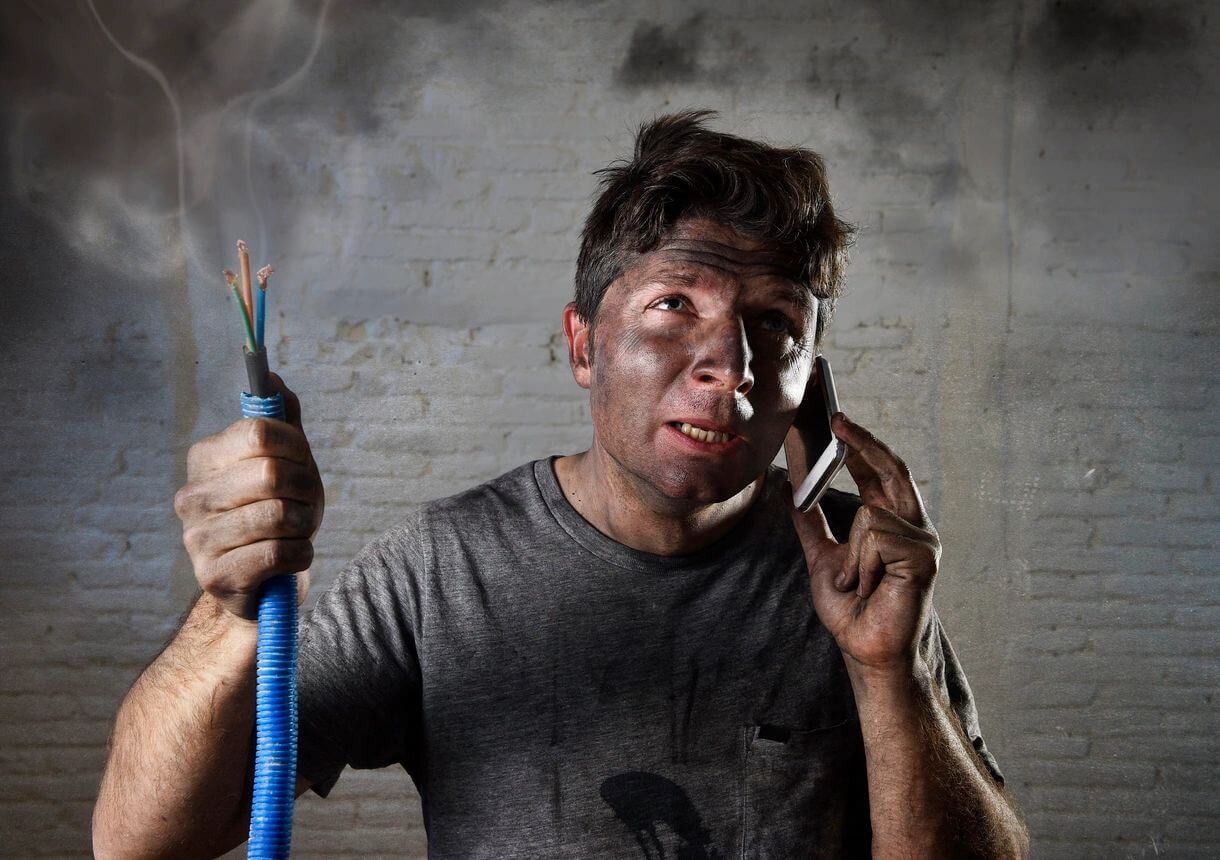
ANOTHER INTEREST LIES IN THE KINETICS OF OSCILLATING AND BISTABLE NON-LINEAR BIOCHEMICAL REACTIONS
Experimental evidence shows that such reactions are very widespread. Yet, it is not even known whether such behavior confers a specific evolutionary advantage on an organism or whether oscillations and bistability are simply unavoidable results of the complex reaction schemes necessary for metabolic control and regulation. We are expanding our interests to include more computationally intensive molecular dynamics simulations of membrane reactions, especially interactions between surfactants and lipid bilayers. Mechanisms of tissue injury in electrical trauma; biophysical control of cellular biosynthetic processes.
Our laboratory's primary efforts are directed at investigating the mechanisms of electrochemical and mechanochemical transduction in cell membranes as they relate to mechanisms of tissue growth and adaptation. We have adopted and refined an in vitro model connective tissue used to study the effect of physiologically relevant mechanical stress on cellular biosynthetic proliferative responses. We have further characterized the mechanical properties and kinetics of the extracellular matrix's remodeling by human fibroblasts in this model connective tissue. Our projects now focus on measuring the importance of frequency and amplitude in governing cellular responses. In the future, we will modify the composition of the extracellular matrix so that transductive electromechanical coupling interactions occur in the extracellular matrix. By controlling this, we will determine the importance of matrix composition in modulating cellular responses.
An important aspect of our research relates to the effects of high-tension electric fields, damaging to cells. We are investigating the effect of supraphysiologic temperatures and supraphysiologic electric fields on the stability of cell membranes. We have established the thresholds for cell membrane breakdown due to strong electric field application and independently, as a result of exposure to supraphysiologic temperatures. We are now investigating mechanisms for stabilizing cell membranes against these effects and possible reseal membranes following membrane permeabilization. This research is also clinically-oriented as it may lead to new therapies for electric shock survivors.